
Layperson Version
It's All About the Genes
Blog #10: Immunogenetics and Immunogenomics (What makes us who we are?)
There’s not enough bandwidth in these blog discussions to address genetics in depth. But a quick review of basic genetics might be valuable here because more than ¾ of our immune system’s functions and regulation is influenced by the 20,000 to 25,000 genes found on the DNA (deoxyribonucleic acid) double helix, packaged into chromosomes in the cell nucleus (Figure 3.1 and 3.2). These chromosomes are identified in a form called a karyotype (Figure 3.3).
Immunogenetics is the branch of science that explores the relationship between the immune system and genetics. Through a number of previous blog discussions we have mentioned T and B lymphocytes and their surface receptors. All of these cells and receptors are genetically produced and programmed from very powerful genes called the Major Histocompatibility Complex or MHC genes. These MHC genes are referred to as “the key to self-recognition” and they are believed to have evolved over 600 million years (see Blog #6).
The chemistry of our DNA and its interactions involved in the making of proteins that make up all the tissue and cells in our bodies is an exquisite process. It is a process that occurs without changing the chemical structure of the DNA through an interaction with our RNA. This extraordinary genetic process is effectively what builds and rebuilds our bodies throughout our lifetime. It also is responsible for how bodies and immune system function, but it is responsible as well for genetic diseases and mutations that produce things like autoimmune disease, cancers, pediatric syndromes and ultimately, the aging process itself.
Included in our understanding of our genetic functions, we must also understand the concept, indeed its foundation, our genome. This is our complete set of DNA, our genes and their interactions between each other, between our environment, and the direct activities of our immune system and every organ system in our body. Specifically, the science of immunogenomics deals with the immense volume of the human genome and its relationship with the human immune system and all its cellular, chemical and biological functions.
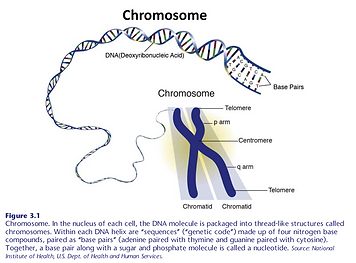

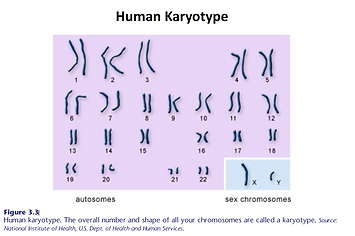
Protein synthesis, the process that produces all of our cells and tissues uses an extraordinarily complex, step-by-step molecular interaction between DNA and RNA which is known as “the central dogma of molecular biology.” It is without a doubt the singularly most important function of our genome and also responsible for the total genes and proteins that make up our immune system. This collection of immune cells, chemicals and functions is referred to as our “immunome.” Mutations in the genes of the genome and immunome are directly or indirectly responsible for all human diseases. Among the approximate 3 billion chromosomes within the nuclei of the cells and their DNA are our 20,000 to 25,000 genes. The number of possible combinations within the chemicals of these genes, or the “genetic codes,” is astronomical. It is among these prodigious numbers of gene combinations that congenital or hereditary, and acquired aberrations or mutations occur. Fortunately, only an infinitesimal amount of them, less than 60 per gene, override a normal cell’s ability to self-destruct when something goes wrong to produce genetic disorders or diseases.
Determining the order of DNA proteins, or their “sequence,” a person’s genetic code is called DNA sequencing and has advanced genetics both for research and clinically. The original sequencing technology took months and even years to record the complete sequence of a person’s DNA. The development of next-generation sequencing (NGS, also called high-throughput or massively parallel sequencing) uses artificial intelligence (AI) and big data analytics. This has sped up the process, now taking only days to weeks to sequence a human genome while dramatically reducing the cost. This is the method now being used to determine the whole genome sequence (WGS) or an even faster method, exome (the coding portion of genes) sequencing. The clinical value of these NGS methods have dramatically demonstrated their value in their use for rapidly identifying coronavirus variants. The original method used to sequence the complete human genome, called the Human Genome Project, to be discussed in the next blog #11) took 20 years to accomplish at a cost of $3 billion. Using NGS, sequencing the complete human genome can now be accomplished in 24 hours for under $500. Now that’s progress!
Blog #11: The Human Genome Project (HGP), Precision medicine, and "AIl of Us"
This blog discussion includes a dynamic combination of genetics, immunology, epidemiology, and public health, the foundational elements of the Human Genome Project (HGP), one of the greatest scientific achievements in history. It was launched in October 1990 and completed in April 2003. The National Human Genome Research Institute (NHGRI) of NIH analyzed random DNA samples taken from 11 donors. Using the older WGS (Whole genome sequencing) method, replaced in 1999 by NGS (Next generation sequencing), it deciphered and mapped the genetic base chemical sequences of 92% of the entire human genetic code, i.e., the genome explained in the previous Blog #10. Subsequently, by March 2022 a group of research scientists, the Telomere-to-Telomere (T2T-CHM13) consortium, completed mapping the remaining 150,000 areas or “gaps” and produced the first truly complete human genome sequence labeled GRCh38. Thanks to the HGP, NGS, and continuing advances in AI and “big data analytics,” the scientific community is now using the examination of chromosomes to identify structural abnormalities and to locate and identify genetic mutations in timeframes of minutes, hours, and days, versus the original “non-AI processes” of weeks, months, even years, if at all. This capability allowed researchers to develop a COVID-19 vaccine in less than a year versus the multiple years and decades it took to develop aa vaccine years ago. It will now also provide rapid identification of viral variants as they developed.
The combination of HGP and NGS technologies has changed the current face of immunology and health care with the capabilities of now locating and identifying mutations. This is dramatically expanding treatment options through immune drug therapies; gene engineering; also modifying procedures that edit and can replace mutations like CAR-T and CRISPR-Cas9 procedures); and stem cell transplantation therapies. All to be exciting treatment modalities are discussed in depth in Blogs #22 through 26. HGP has also led to a new mapping initiative of the immunome, remember, the immune system’s genome? Using a mouse model initially, the Immunological Genome Project (ImmGen) has begun the process of mapping gene expression and how it regulates the immune system.
Complementing the immunologic technology advances being witnessed through HGP, we are also witnessing the introduction of a new level of real personalized, “precision medicine” and preventive health care. This new level of health care will be able to identify and correct gene abnormalities in many instances before they produce negative effects. The concept of precision medicine, aka personalized medicine, has become a significant part of human genetics. It is indirectly related to immunology through its enormous potential value in health care. The concept provides profound applications and impact on the future of care including the diagnosis, treatment, and potential cure and prevention of all diseases.
Precision medicine is a concept that uses genetics as its foundation. According to the CDC’s (Center for Disease Control) “Precision Medicine Initiative,” this relatively new term takes into account variability in a person’s genes, their environment, and lifestyle. It allows doctors and researchers to predict more accurately treatment and prevention strategies for a particular disease in specific patients and groups of patients. This will replace the “one-size-fits-all approach” to healthcare. Its goal is to find unique disease risks and treatments that work best for individual patients. It includes the use of family history, screening for diseases before you get sick; tailoring preventive strategies for patients and populations; tailoring specific treatment modalities for individuals based on their genetic code; and considering a patient’s overall DNA profile to structure a personalized health care plan with an overall goal of health prevention rather the traditional disease model.
As part of Precision Medicine, an initiative by the National Institute of Health (NIH) entitled “All of Us” will be tracking the history, physical findings, genetics, behavioral, and environmental factors of one million Americans for several years. They will be assessing health factors; developing healthcare solutions that make the best decisions to prevent or treat disease; predict epidemics; and improve the quality of life. This ambitious concept combines medicine, biology, genetics, statistics, immunology, and AI computing to create large-scale biobanks of complete genome-sequenced and phenotype information from hundreds of thousands of people. It promotes the idea that everyone should have a DNA profile on file. A great idea!
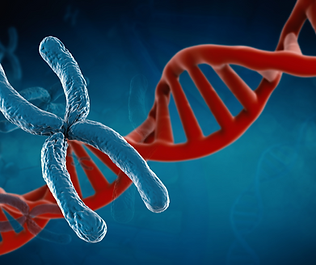
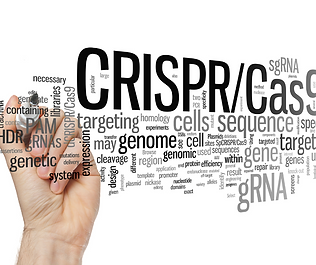
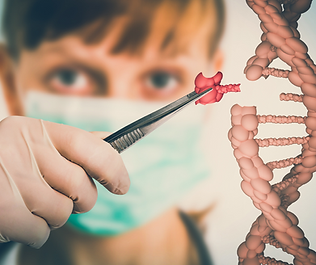
Blog #12: XCI (lyonization)
A process of immense importance in immunology and immunogenomics that involves genetics and embryology is the interesting phenomenon of X chromosome inactivation or XCI. It represents numerous immunologic paradoxes and is a phenomenon we discuss numerous times throughout the book because it effects so many aspects of immunology. This blog will “attempt to explain” the basic embryology and more so, the genetic considerations involved in XCI and they all will be revisited with some frequency in subsequent blog discussions.
Among the 46 paired chromosomes in each of the cell in our body, the 23rd pair is called the sex chromosome. Females have 2 X chromosomes as their pairings, while males have one X and one Y chromosome as described in Blog #10. XCI, sometimes termed “lyonization,” named after the British geneticist, Mary Lyon who hypothesized the theory in 1961, proposes that within 5 to 6 days before preimplantation of the embryo, one of the female’s X chromosomes is randomly and permanently inactivated or genetically “silenced” in most, but not all of her cells, with the exception of her lifelong reproductive egg cells which remain with 2 X chromosomes. The XCI process prevents female cells from having twice as many gene products from the X chromosomes as males. It provides the increased potential in females for double protection against certain diseases. But it also introduces the increased risk of negative immunogenic influences (another immune paradox). Add to this the overwhelming abundance of a unique a RNA molecule called microRNA or miRNA on the X chromosome and we have one of the most significant paradoxes of the immune system.
The X-chromosome has approximately 155 million base pairs in their DNA which translate to about 900-1400 genes that account for about 5% of the total DNA of a cell versus the Y chromosome at about 70 genes and carries about 58 million base pairs or about 2% of the total DNA of a cell. The protein synthesis we discussed back in Blog #10 of these genes will have dramatic implications in the development of the female’s physical traits, her immune system, risk of autoimmune diseases, and cancers, all to be described in further detail in their respective blogs. The woman’s cells can express X chromosome genes differently (and randomly) from each other. This is gives females more diversity than males. Remember, after XCI, the female has more X chromosomes than the male, meaning that females have more genetic options during development and thus, more ways to prevent disease. Again the bias of females versus males from Blog #4.
Diseases caused by mutations in genes on the X chromosome are called X-linked diseases, that is, genetic diseases in which the disease-causing gene exists on the X chromosome. Males do not experience XCI because they only have one X chromosome. So, if males have a disease-causing gene on their X chromosome, it will more likely be active and increase the risk of causing an “X-linked” disease. Conversely, XCI helps protect females from X-linked diseases but, given the combinations and permutations created by 900-1400 X chromosome genes, the female is not entirely excluded from their possibility, though rare, as in Rett disease, Turner syndrome, etc..
That MicroRNA or miRNA we mentioned in the first paragraph is a noncoding RNA gene meaning it is not involved in protein synthesis process. It is abundant on the female X chromosome which contains 10% of all miRNAs in the human genome. Estrogens regulate miRNA and further validate a compelling explanation for female predilection towards autoimmune diseases. It is suggested that about 15% of genes escape the XCI process, all of which recent research has confirmed as primary attributions to the female bias of autoimmune diseases, especially SLE. These “escapees” also play a significant role in oncogenesis or cancer production which we will be discussing in Blog #28.
Blog #13:The Microbiome: "Gut-axis-du jour"
It’s a challenge to find an appropriate place to introduce the microbiome in a discussion where you’re trying to develop an evolving narrative of the immune system. As you will see, it is so intimately involved in so many aspects of the system that you can introduce it in a dozen places. The slightly silly subtitle I added, "gut-axis-du jour," ain't so silly when you follow the microbiome research and literature. I used more than 2400 words discussing it in my "Paradox of the Immune System" book and that only "tree-topped" it up to June 2022. Not a week goes by that there isn't another update on the microbiome,
What we’ll do in this brief discussion is introduce its basic elements and then expand on its relevance in subsequent blog discussions as it keeps resurfacing and applies as an important feature in so many immune topics. And, it works well to start here, just after our genetics and genomics discussion back in Blog #10 because the microbiome is referred to as “the second genome” of the human body.
Starting with a little trivia about the microbiome, there are more bacteria in our body (greater than 30 trillion) than there are human cells with an estimate of approximately 57% of our cells actually being a mixture of microbes and 43% human cells. It is this enormous array of microbiota, aka microorganisms found in a particular habitat, in this case our body, that makes it “the second genome.” The unique difference in the human versus the microbial genome is their evolutionary development. Whereas the DNA of the human genome is very stable allowing for predictable heredity with only slight mutations over time, sometimes over millions of years, the microbial “second” genome” changes far more frequently. As a matter of fact, the only time it is predictable is at birth. That is when the newborn will inherit some microbes from the mother described in Blog #8. As we described, this is a critical process that allows the baby to begin to deal with pathogens outside of the womb. The transfer of microbes to the newborn happens during vaginal births when the baby is exposed to the mother’s gut and vaginal microbes during delivery. This triggers the baby’s immune system, allowing for early protection and more so, a better ability to absorb nutrients from the mother’s breast milk.
Everything in this world is covered by microbes, so obviously we are constantly inhaling and ingesting microbes and creating our own gut microbiota. And so, the theory is that the constant 24/7 exposure of gut microbes to immune cells, from birth on creates a state of chronic, albeit subclinical most of the time, inflammation in our gastrointestinal (GI or gut) system from oral to colorectal syndromes. This chronic state produces a “symbiosis” or a “biological synergy” of “peaceful and complementary coexistence” between immune cells and the microbes. Such a biological synergy includes fighting off invading microorganisms like pathogens, thanks to that great antibody-encoding gene we’ve mentioned and described a number of times as part of our human genome. Our immune system has an almost infinite array of antibodies to achieve this goal, but because of the enormity of the task, it looks like we have evolutionarily come to enlist help from “normal flora” microbes or “the microbiome.” No doubt, an imbalance of this powerful microbiome can produce serious health consequences.
The microbiome also involves yet another specialized subset of the T cells that we have mentioned previous, and a rather powerful T cell at that. As discussed previously in Blog #5, it is the T regulatory cell or Treg, also referred to as the T suppressor or Ts cell. One of its functions is to suppress the immune system when it becomes too overactive and dysregulated. It has been shown that bacteria from the microbiome trigger Treg cells (and other killer immune cells like natural killer cells [NKs] and T cytotoxic [TC] cells). This causes the microbiome to become a mitigating force against inflammation, autoimmune disease, and even cancers as it modulates the immune system. Human’s inherent desire to “clean” everything, called the “hygiene hypothesis” suggests that we may be compromising our microbiome. Could this be a cause of increasing autoimmune diseases and some cancers? And regarding cancers and the microbiome, distinct aspects of the microbiota have been reported to have either pro- or antitumor effects. Thus, modulating the microbiome in certain individuals could also have implications for cancer prevention. More on this in Blog #29.
This is the last blog discussion related to “Our friendly immune system” or Section 1 of the book, “The Paradox of the Immune System.” Section 2, entitled “The enemy within us” will deal with the darker side of immunology. To wit, I used a “strained” metaphor in the book which I’ll try here as well. “Having paid a peaceful, relatively untroubled visit to “our friend,” the regulated immune system in Section 1, now it’s time to visit the darker, far less pleasant side of immunity, the “dysregulated” immune system. I’m afraid “we’re not in Kansas anymore, Toto. It’s time to ‘look behind the curtain’.” Sorry about that.